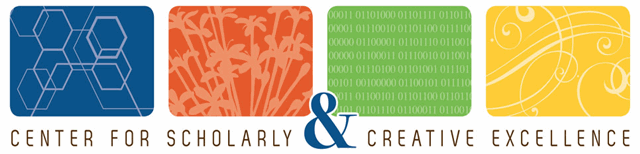
Faculty Scholarly Dissemination Grants
Mechanotransduction in Mouse Models The Effect of Mechanical Loading on the Ulna
Department
School of Engineering
College
Padnos College of Engineering and Computing
Date Range
2013-2014
Disciplines
Engineering
Abstract
Introduction: Osteoporosis is a commonly observed medical condition in aging populations which results in an increased risk of skeletal fractures due to accelerated bone loss. Exercise has been suggested as a possible method for slowing the effects of aging on bone loss. The phenomenon of load induced bone growth is known as mechanotransduction. Obtaining a better understanding of the variables that affect mechanotransduction such as loading rate, loading frequency, loading magnitude, and rest between loading bouts allows a more effective exercise regimen to be created for patients with osteoporosis. This study utilized mouse models to explore the effects of mechanical loading on the ulna. Various loading schedules were executed and the resulting changes in mechanical properties of loaded bone were compared to the non-loaded bone. Materials and Methods: Utilizing a material testing apparatus, anesthetized mice underwent cyclical compressive loading on the right forelimb for predetermined periods of time. The number and frequency of loading bouts were determined based on prior published research (Robling et al. 2002). There were three groups of mice that were sacrificed at 15 weeks (No Loading: N=8, Three Loading Bouts for One Week: N=9, Three Loading Bouts for Three Weeks: N=4). There were two groups of mice that were sacrificed at 18 weeks (No Loading: N=6, Three Loading Bouts for Three Weeks, N=6). The mice were sacrificed at completion of the loading schedule and both forelimbs were harvested. MicroCT (µCT) imaging (µCT: Skyscan, Kontich, Belgium) was used to provide cross sectional images of each ulna. CTAn (µCT: Skyscan, Kontich, Belgium) and BoneJ (Bethesda, Maryland) software was used to calculate the cross sectional area along the length of the bone, as well as the minimum and maximum moment of inertia. Comparisons were made between loaded and non-loaded bone for each mouse, as well as comparisons between the various loading schedules. Results and Discussion: Among the three groups of mice that were sacrificed at 15 weeks (No Loading, Three Loading Bouts for One Week, Three Loading Bouts for Three Weeks), there was a trend toward increased bone apposition in the loaded limb compared to the non-loaded limb at the midshaft (3% greater cross sectional area in loaded ulna compared to non-loaded ulna). However, this increase was not determined to be statistically significant. For the two loading groups of mice that were sacrificed at 18 weeks, there was a significant difference in the amount of bone growth observed in the loaded limb compared to the non-loaded limb at the midshaft (16% greater cross sectional area in loaded ulna compared to non-loaded ulna). Further analysis of the µCT data from the mice in the 18 week old age group showed increased bone apposition distal to the midshaft of the ulna in the loaded limbs. There was also an increase in the minimum moment of inertia both distal and proximal to the midshaft. The distal increase was due to increased cross sectional area, while the proximal increase was due to a change in the geometric shape of the bone. Conclusions: Application of compressive forces to bone increases the rate of bone apposition and results in a larger cross sectional area of the bone. Depending on the bone geometry and the nature of the forces experienced by the bone, varying amounts of growth are initiated. By understanding how force impacts bone growth, exercise regimens aimed at optimizing bone apposition can be created. Future work may include analysis of the cellular signaling pathways that are activated as a result of mechanical loading and the creation of therapeutics that can modulate the response of bone to mechanical loading. Acknowledgements: Special thanks to the Van Andel Institute and specifically to Bart Williams, PhD for allowing this research to be performed in his lab. Also, thank you to the members of the Williams lab for their assistance. Thank you to Phil Boonstra, PhD from the University of Michigan Cancer Center for providing statistical consulting, advice, and analysis of the µCT data. Lastly, thank you to the National Science Foundation for supporting this research through a grant under the American Recovery and Reinvestment act of 2009 presented to Dr. Samhita Rhodes and Dr. John Farris.
Conference Name
Biomedical Engineering Society Annual Meeting
Conference Location
Seattle, WA
ScholarWorks Citation
Ashby, Blake; Moren, A. Vander; Hotaling, H.; Williams, B.O.; and Burgers, T.A., "Mechanotransduction in Mouse Models The Effect of Mechanical Loading on the Ulna" (2014). Faculty Scholarly Dissemination Grants. 984.
https://scholarworks.gvsu.edu/fsdg/984